Measurement of the 3D position and orientation of single molecules
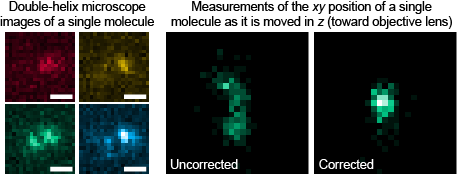
M. P. Backlund*, M. D. Lew*, A. S. Backer, S. J. Sahl, G. Grover, A. Agrawal, R. Piestun, and W. E. Moerner, "Simultaneous, accurate measurement of the 3D position and orientation of single molecules," Proc. Natl. Acad. Sci. USA 109, 19087 (2012). [Highlight in Nat. Methods, Journal, PDF]
Project summary video
"Measuring the 3D position and orientation of single molecules simultaneously and accurately with the double helix microscope," OSA CLEO: 2013, San Jose, CA, June 2013. [Conference abstract]
Introduction
In January 2011, my team and I received an e-mail from a prospective postdoctoral associate visiting our lab:
"I don't know to what extent you're aware of the recent article I attach, which has very important implications. Let's discuss at some point – I would be interested to see how robust the DH [double-helix microscope] approach is in this respect, in case you have considered orientation effects so far."
Little did we know, this e-mail and the paper it refers to would spur a project that would last almost two years. In the intervening time, we sent countless e-mails back and forth, scoured the literature, and had numerous brainstorming sessions about how to solve what we lovingly called, "the problem." This is an account of the saga surrounding this project.
My research uses single molecules to decorate the biological structures we're interested in looking at. These molecules act as reporters of where the structures are within living cells, like light bulbs decorating a Christmas tree. When the molecules emit light through fluorescence, our microscope images that light onto a camera, and we can record the position of each molecule with very high precision. If all of the molecules are lit at the same time, they will blur together, and we'll see a blurry silhouette of the Christmas tree. However, if these molecules blink, we can localize each molecule as it blinks on and reconstruct the Christmas tree point by point, bulb by bulb, molecule by molecule. The resulting reconstructed image has much higher resolution, hence the term super-resolution microscopy.
The paper that my colleague alerted us to discusses a possible source of large errors in our reconstructed images. Even though a molecule may appear to be at a certain position on our camera, it isn't actually there because its image is skewed by a phenomenon we came to call the "dipole shift" effect. Single molecules are not point sources (they're actually oscillating electric dipoles), so the light they emit is bent by the microscope in a particular way: If we use a camera to measure the position of the molecule, we could make errors up to ~150 nm, which totally swamps our claimed precision of ~10-30 nm. What good can our microscope be with this kind of error?
Importance
This project, for the first time, demonstrates that the three-dimensional position and orientation of single molecules can be measured simultaneously from snapshots taken by a microscope. This means that one can know all there is to know about the spatial properties of the molecules in a given sample. A scientist can take a look at a signaling protein in a cell, measure how it moves and tumbles towards its destination, and demystify the vast complicated cellular machinery at the heart of life itself. Moreover, since we can measure position and orientation simultaneously and accurately, we do not have to worry about the "dipole shift" effect.
Tasks completed
- We simulated how light from an oscillating electric dipole is collected by a microscope objective lens and relayed onto a camera, taking electric field polarization, wave diffraction, and reflection/refraction at a mismatched interface into account.
- We simulated how this light interacts with the double-helix microscope and how dipole orientation affects the images we see on a camera.
- We built and aligned a pyramidal mirror-based 4f optical setup utilizing a reflective liquid-crystal spatial light modulator placed in the Fourier plane.
- We learned how to make single-molecule samples whose fluorophores were well-separated, fixed in both position and orientation, and stably emitted millions of photons before photobleaching.
- We carefully matched experimentally measured single-molecule images with those predicted by theory to measure 3D position and orientation of single molecules with our microscope.
- Our article was published in Proc. Natl. Acad. Sci. USA, featured as a research highlight in Nat. Methods, and presented at several conferences.
Skills gained
- Theory and simulation of vectorial diffraction of electromagnetic fields
- Design, building, and precise alignment of a 3D optical setup, with light being reflected vertically out of the plane of the optical table
- Careful measurement and analysis of single-molecule fluorescence images. We designed and performed many experimental controls to ensure both precision and accuracy
Lessons learned
- The project team makes all of the difference. We all contributed to the brainstorming and struggle to figure out the source of "the problem" and how to fix it. This was truly a team effort that would not have succeeded without teamwork.
- Precise modelling and theory can really be verified in real-world experiments. Without our detailed mathematical models of electromagnetic waves, we would not have been able to quantitatively solve "the problem." Intuition is valuable, but only precise and accurate math will prove that you know what you're doing.
- Careful attention to detail is valuable when trying to carry out precision science. Working with such a detail-oriented team showed me how successful one can be working on an intricate project like this one. There are projects that are proofs of principle; you can just throw something on the microscope and have everything work out. In this project, we needed to be absolutely methodical in making sure that our measurements, conclusions, and calculations were absolutely 100% correct.
Impact
This project proves that single-molecule based imaging techniques are not doomed to failure by the "dipole shift problem." However, this is a complicated solution to a complicated problem, a problem whose severity is unknown when imaging biological samples. This work could inspire optical methods designed to probe orientations or dipole shift errors of single molecules directly. This type of "point spread function engineering" could radically change the conventional workhorse of the biological laboratory.
Related work:
- M. D. Lew*, M. P. Backlund*, and W. E. Moerner, "Rotational mobility of single molecules affects localization accuracy in super-resolution fluorescence microscopy," Nano Lett. (published online Jan. 29, 2013). [Journal]
- A. S. Backer, M. P. Backlund, M. D. Lew, and W. E. Moerner, "Single-molecule orientation measurements with a quadrated pupil," Opt. Lett. 38, 1521 (2013). [Journal]